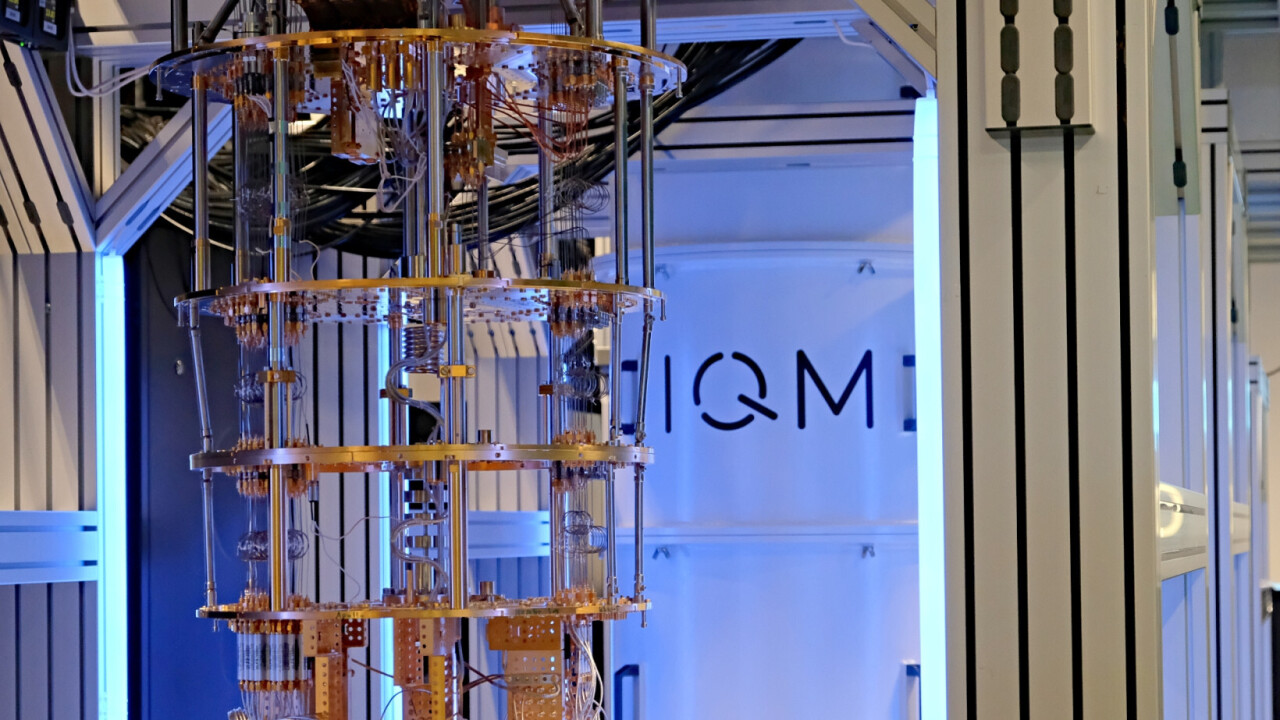
“The Future is Here,” declares a glowing neon sign at the entrance to IQM’s quantum data centre in Munich. It’s a bold claim — but one the Finland-based startup is determined to fulfil.
To the right of the entrance sign stands a hefty, metal blue door. My host, physicist Frank Deppe, IQM’s head of quantum processing unit (QPU) technology, ushers me inside.
Opened last year as part of IQM’s European expansion, the facility hosts six state-of-the-art superconducting quantum computers — used for the company’s own research and offered as a cloud-based service to scientists around the globe.
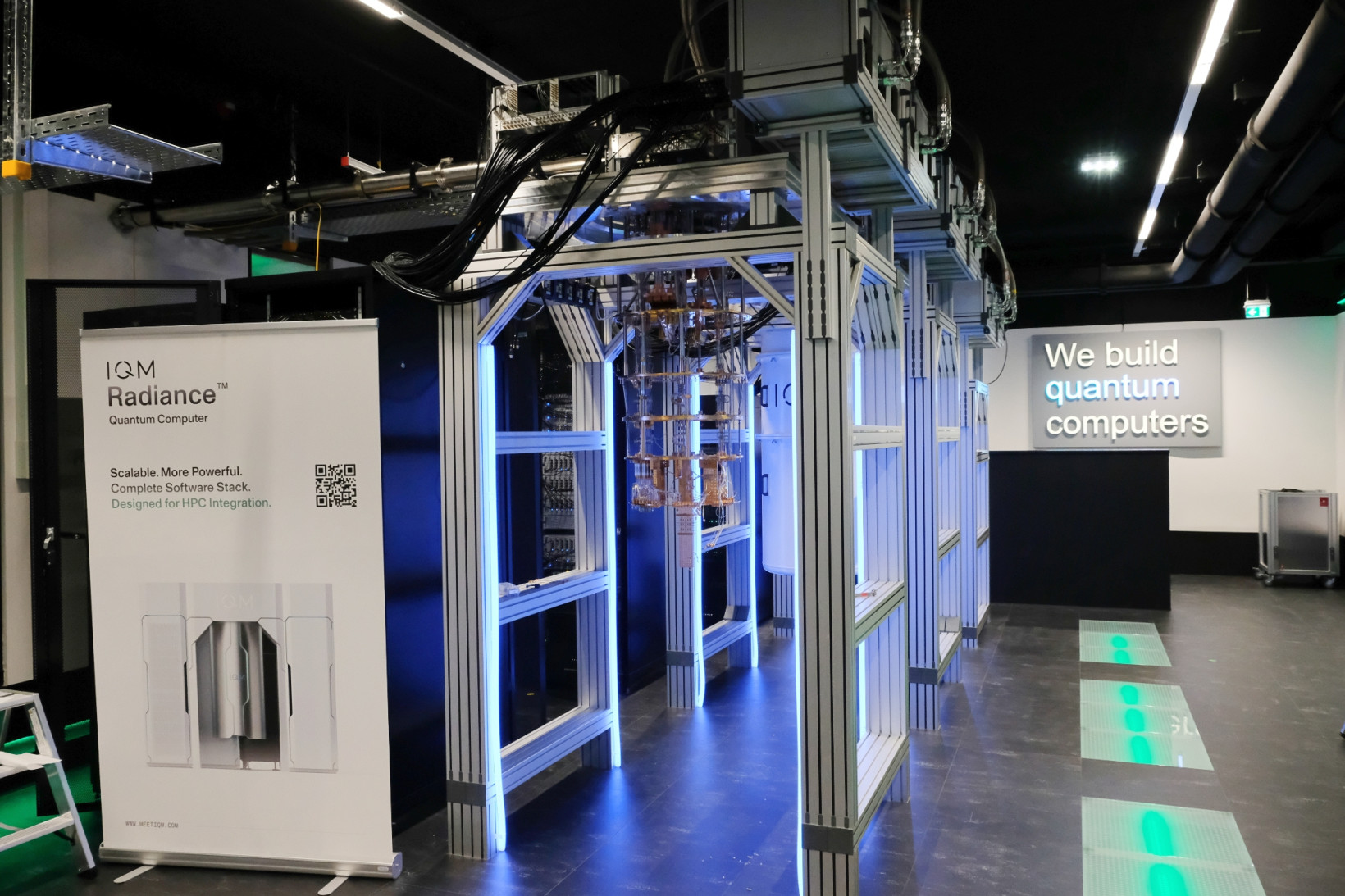
My initial impression is the sound — a low, steady purr punctuated by a bizarre rhythmic pumping noise. That, I would later discover, was the heartbeat of a quantum computer.
The centrepiece of the data centre, though, is the cryostats — the golden chandelier-like structures that have become synonymous with quantum computing in the public imagination.
Cryostats are made up of an intricate system of gold-plated brass and copper wiring that channels microwave signals down to the QPU or “chip,” which sits right at the bottom of the chandelier. These microwave pulses allow scientists to control and manipulate the qubits on the chip, and, in turn, run algorithms to perform quantum calculations.
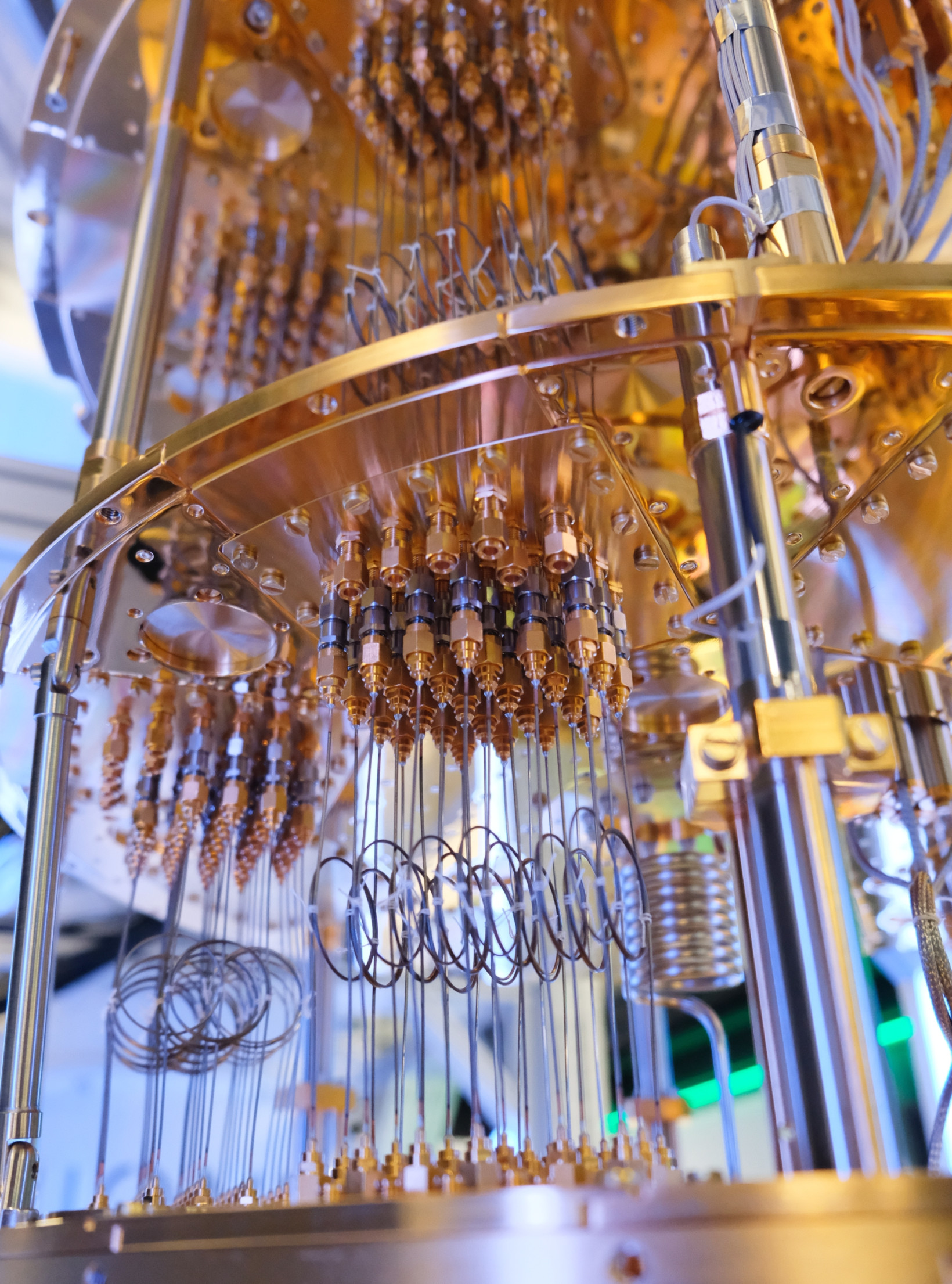
For all this to work, however, superconducting quantum computers need to be cooled to close to absolute zero (or -273.15 degrees Celsius). That makes machines like these among the coldest places in the known universe.
Qubits, which are the basic units of information in a quantum computer, are incredibly sensitive — to heat, vibration, stray particles, or electromagnetic signals. Even the slightest disturbance can cause errors or wipe out information entirely, says Frank, gesturing around us as if he can see the waves and particles flying around the room.
At ultra-cold temperatures, however, superconducting materials lose all electrical resistance, allowing qubits to maintain their delicate quantum properties. But ultra-cold isn’t enough — qubits also need near-perfect isolation from other particles in the air. That’s why cryostats are placed in a thick metal vacuum chamber, which helps to shield the qubits from interference.
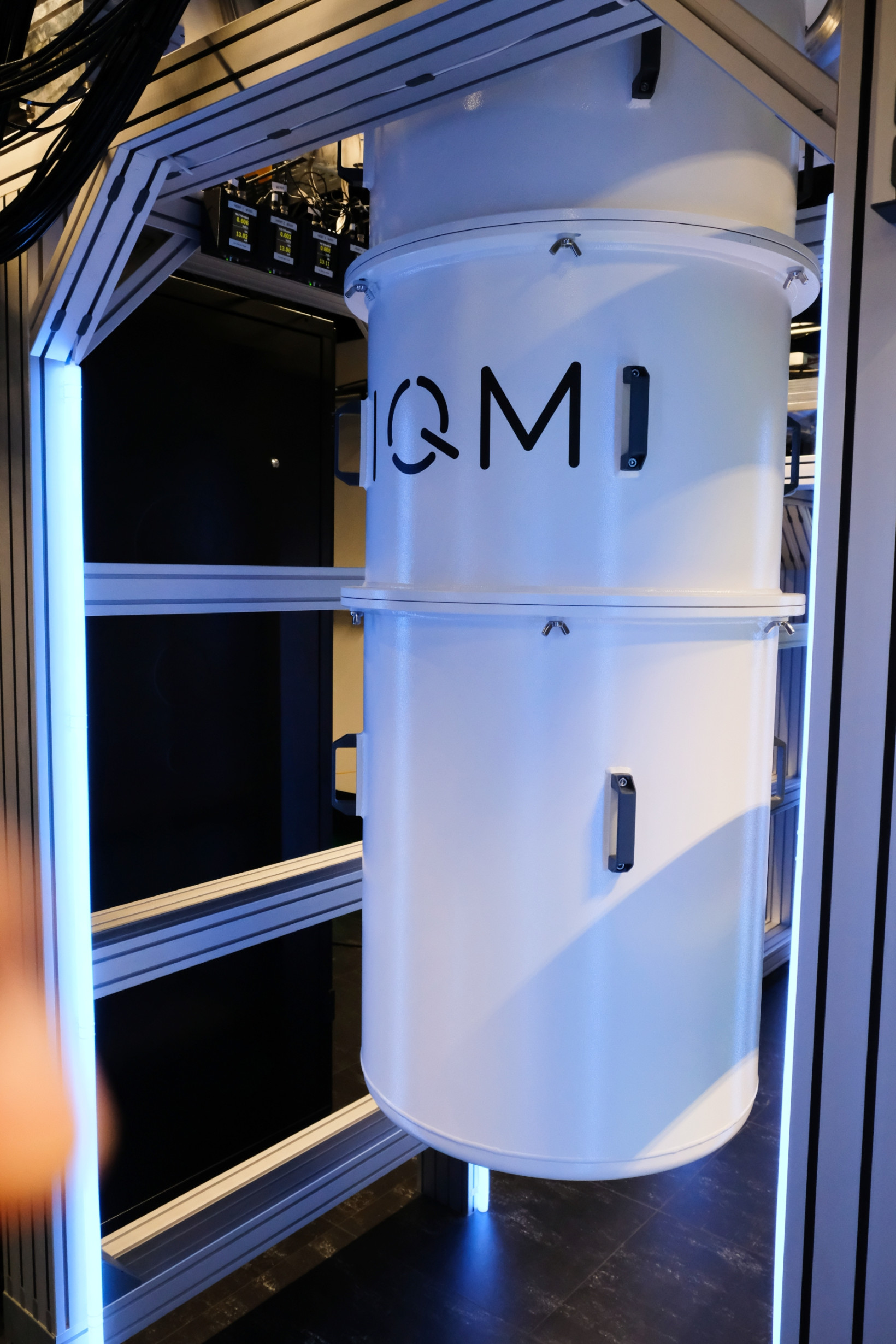
Each machine is supported by some serious industrial hardware. One of the largest pieces of equipment in the lab is the cryogenics system. Comprising a network of compressors, tanks, pumps, and pipes, its job is to transfer liquid helium to super-cool the cryostat. The helium compressor produces the distinctive rhythmic sound of a quantum computer — the cryostat itself is completely silent.
Then there are the servers, placed beside each cryostat. They provide the precise control and support infrastructure that allows delicate quantum systems to operate effectively. They also produce the specific microwave pulses required to keep the qubits stable.
Yes, even the quantum computers of the future will need classical computers to function, Frank says.
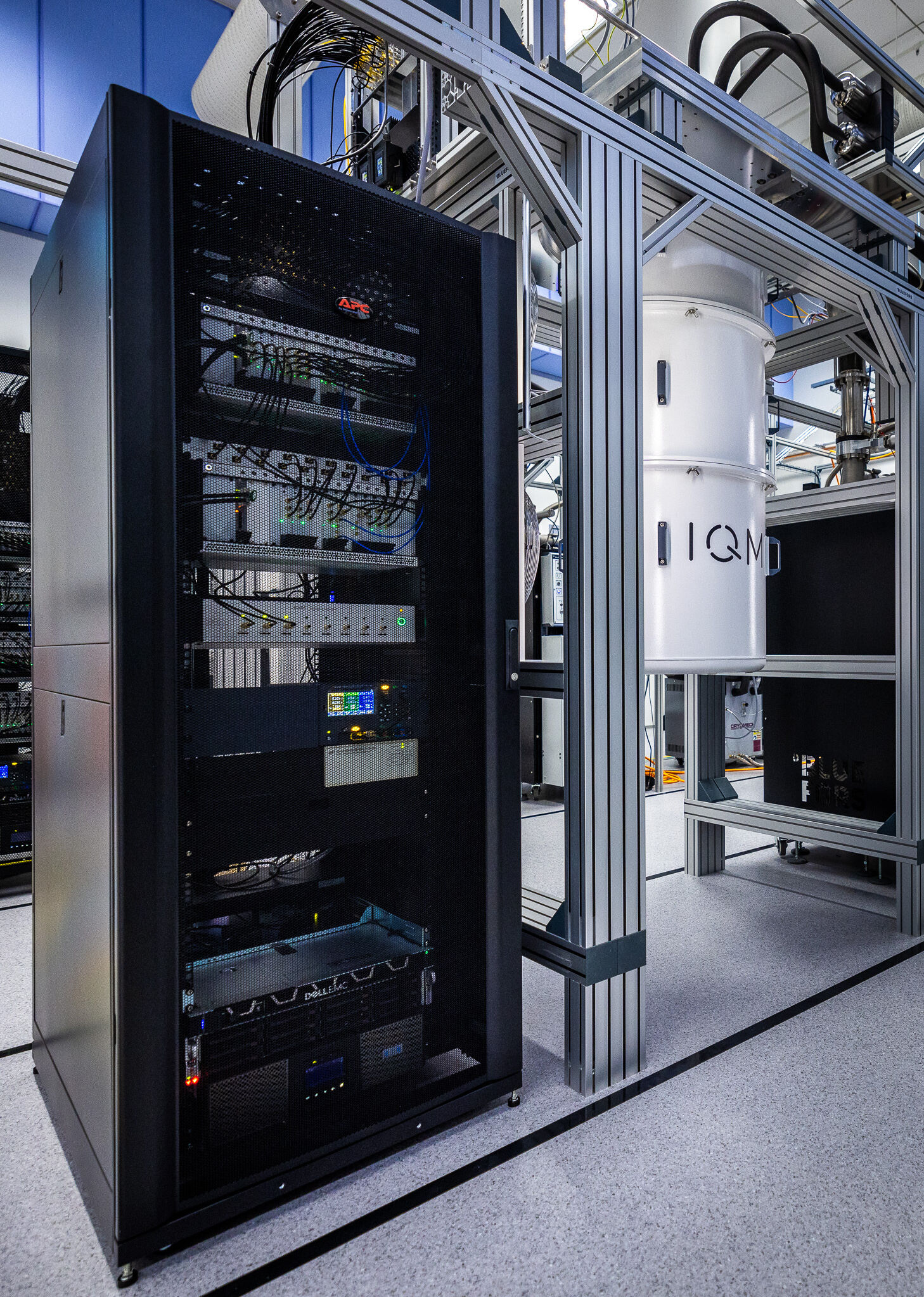
I was amazed by the extraordinary amount of infrastructure needed to power a quantum chip barely larger than my fingernail. But all that tech is essential — it protects the fragile qubits while still allowing for their manipulation.
“You need to isolate qubits from the environment — but still control them,” says Frank. “That’s the engineering paradox of quantum computing.”
Tapping into the subatomic world of quantum mechanics — with phenomena such as superposition and entanglement — to perform useful calculations is one of the toughest challenges in modern science. It’s baffled researchers for decades. But now, after years of steady progress, we’re closer than ever to potentially world-changing applications — and the payoffs could be huge.
Towards quantum advantage
The quantum computers of the future are expected to solve problems that are far beyond the reach of today’s most powerful supercomputers — a point known as “quantum advantage.” These machines could simulate complex molecules for drug discovery, design new materials from the atomic level up, and revolutionise logistics and finance by cracking massive optimisation problems. They could also break all internet encryption on what is known as Q-Day — so there are risks, too.
However, most experts agree that we’ll need a 1 million-qubit system and beyond to make those sorts of calculations — and that’s still a long way off.
We’re currently in what is known as the Noisy Intermediate-Scale Quantum (NISQ) era, where we have small quantum computers that can run real experiments but are still too “noisy” and error-prone to do anything truly groundbreaking.
IQM’s quantum processors currently range from six to 50 qubits. Next year, it’s set to release a larger 54 to 150-qubit system called Radiance, which it says will “pave the way” to quantum advantage — when a quantum computer can solve a problem no classical computer can). The company hopes to produce a 1 million-qubit system by 2033.
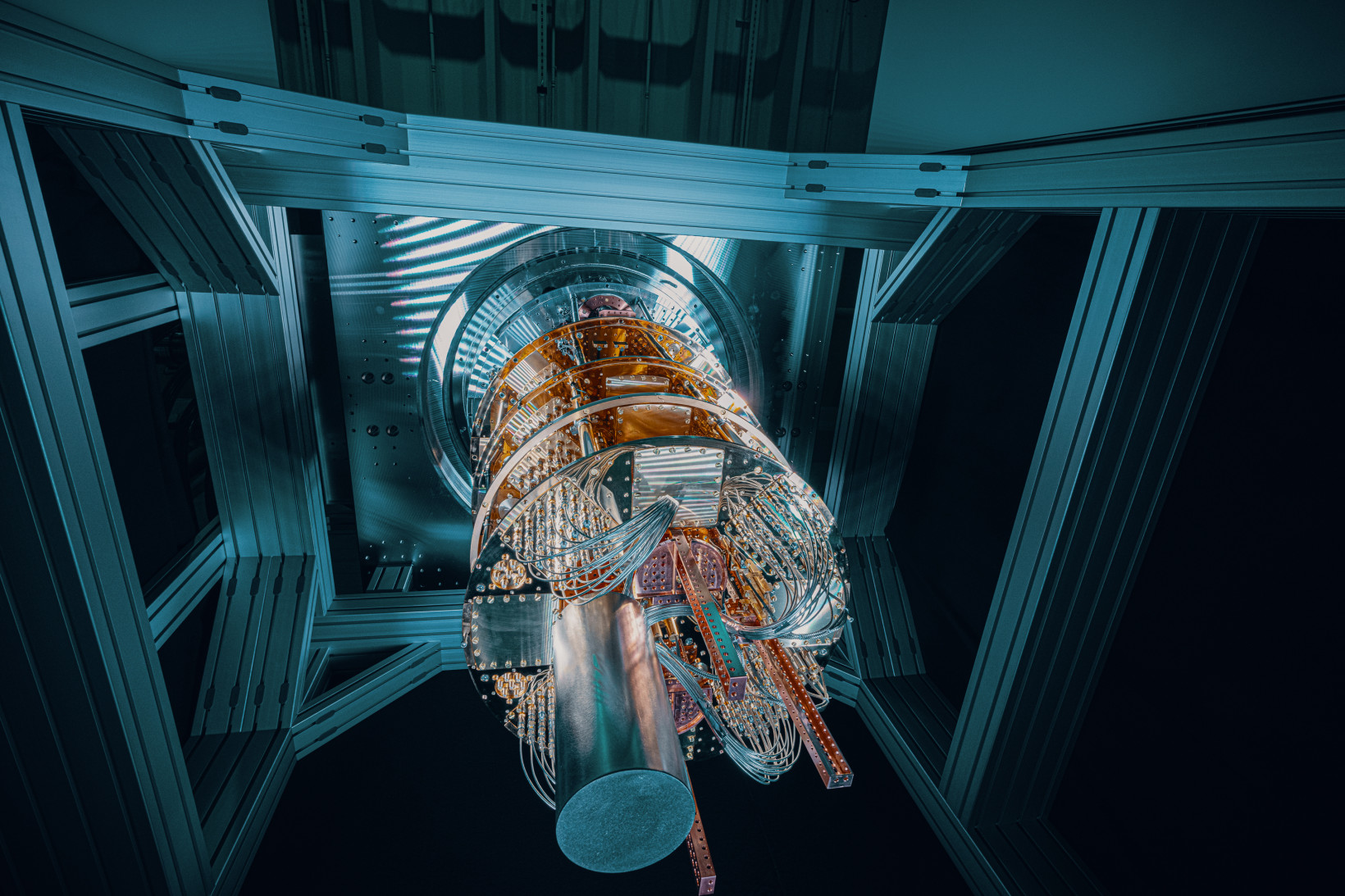
Headquartered in Helsinki, IQM has built a business based on helping researchers train on and navigate smaller systems before larger ones become commercially available. Using these machines, scientists can already explore quantum algorithms, develop hardware, and prototype solutions for specific problems such as climate modelling or drug discovery.
Founded in 2018, IQM has raised $210mn to date, making it Europe’s second best-funded quantum computing company. According to Bloomberg, the startup is also in talks to raise over $200mn in fresh capital, which would bring its total to over $400mn. In June, the company’s co-founder and CEO, Jan Goetz, will share his vision of Europe’s quantum future at TNW Conference.
Located in Finland’s thriving quantum startup ecosystem, IQM has built over 30 full-stack quantum computers to date at its facility in Espoo, west of the capital, Helsinki. This site also houses Europe’s only private quantum chip factory.
Inés De Vega, vice president of innovation at IQM, tells TNW that its quantum processors have “similar, if not better, performance in terms of fidelities” than IBM, often considered the world leader in quantum technology. Fidelity refers to the accuracy with which a quantum computer can perform operations on qubits without introducing errors — a critical metric for building reliable and scalable quantum systems.
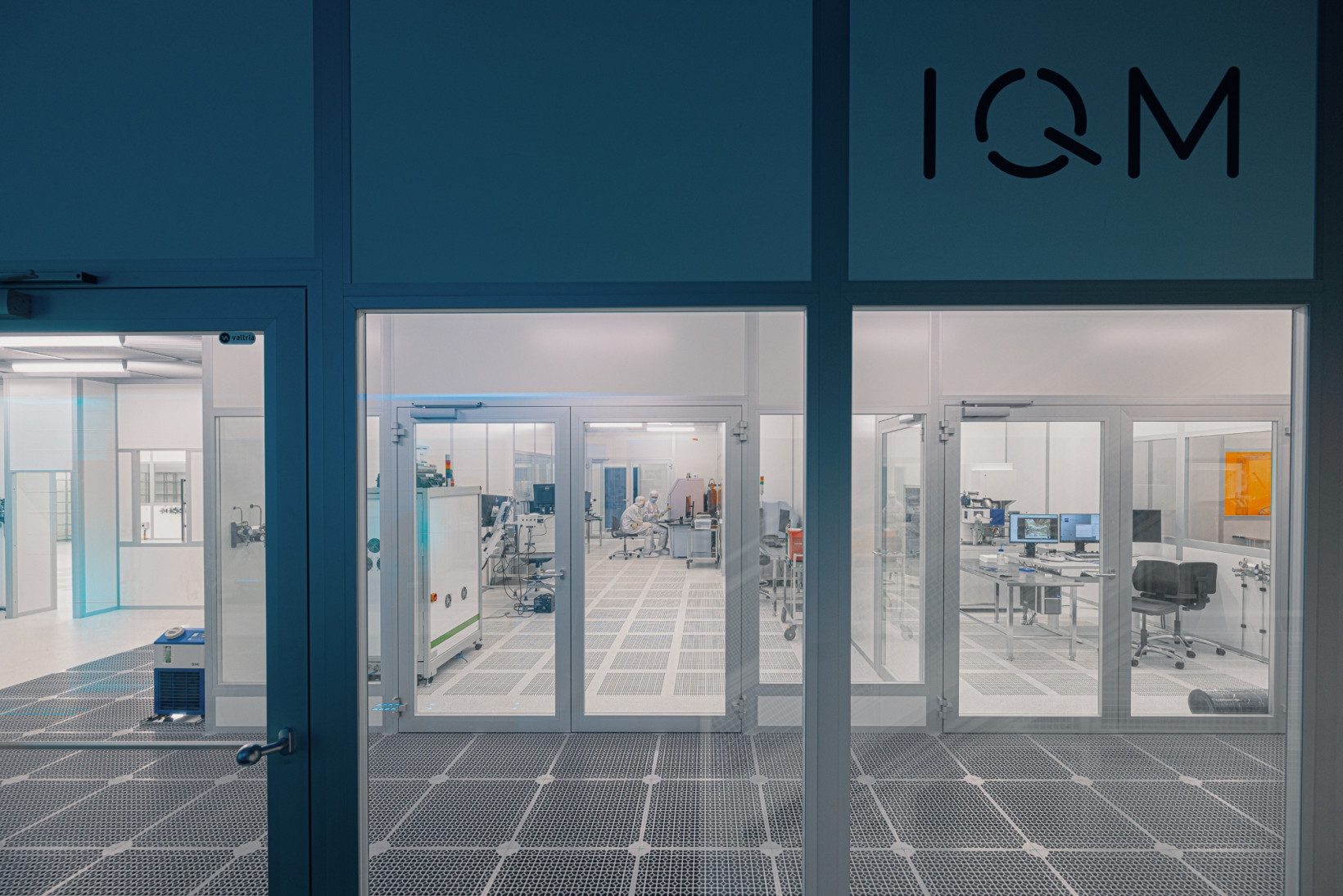
While IQM is one of Europe’s most prominent quantum startups, it’s far from alone. There are currently 122 quantum computing companies on the continent, with a combined value of almost $13bn, according to Dealroom data.
UK-based Quantinuum is the best-funded, having raised $647 million at a $5bn valuation. Instead of using super-cooled superconducting circuits, Quantinuum develops trapped-ion quantum computers, which use electrically charged atoms controlled by lasers for qubits. Other European big shots include French startup Pasqal and the UK’s Oxford Quantum Circuits.
In the US, tech giants such as IBM, Google, Amazon, Microsoft, and Intel, plus well-funded startups like PsiQuantum, are all racing to scale up their own quantum computers and reduce error rates.
Globally, more than 30 governments have pledged over $40bn in public funding for quantum technologies, set to be deployed over the next decade.
Both the private and public sectors are chasing the holy grail: a fault-tolerant quantum computer — one powerful and stable enough to run complex algorithms with minimal errors. IQM aims to get there by 2030, according to its publicly available roadmap.
IQM’s estimate is on the optimistic side. In February, Google’s CEO Sundar Pichai said he believes “practically useful” quantum computers are five-to-10 years away. A month earlier, Nvidia’s Jensen Huang suggested we’re still at least 15 years out — a comment that sent quantum stocks tumbling.
Truth is, no one knows exactly when we’ll get there. But one thing is clear: reaching the quantum finish line will demand years of experimentation, iteration, and engineering breakthroughs. That work is already underway in labs such as IQM’s, where the boundaries of physics are being pushed, one qubit at a time.
At TNW Conference on June 19, IQM CEO and co-founder Jan Goetz will join Elvira Shishenina, senior director at Quantinuum, and Tom Henriksson, general partner at OpenOcean, for a panel discussion titled “Quantum Race: Can Europe Secure Leadership in Quantum?” Tickets for the event are now on sale. Use the code TNWXMEDIA2025 at the check-out to get 30% off the price tag.
Get the TNW newsletter
Get the most important tech news in your inbox each week.